Preventing Fretting Corrosion of Orthopedic Devices

By Engaged Expert
Maciej JakuckiMaciej Jakucki has performed and managed a wide variety of medical testing projects and programs to meet FDA and CE requirements.
While implantation of orthopedic devices often brings relief to an existing medical condition, secondary failure due to corrosion and resulting corrosion products can reverse positive outcomes.
Biocompatibility testing helps ensure that materials, including stainless steel, PEEK (polyether ether ketone), polyethylene, titanium, and cobalt chrome, are safe for implantation. However, as these materials begin to interact with the human physiological environment over time, the interaction can result in accelerated failure or disassociation of the implants, rejection of the implant by the immune system, or poisoning by elevated metal ion levels in the body.
“Fretting Corrosion” is of particular concern in multi-component devices. When two components are in contact with each other, cyclic loading can cause micromotion between the mating surfaces. This oscillatory motion can cause localized deformation, material removal, and transfer known as fretting. Environmental characteristics including elevated temperature, an electrolytic fluid medium with non-neutral pH, and differences in material chemistry can increase the chances of chemical attack, or corrosion, on one or both of the surfaces. The fretting and corrosion compound each other leading to the term, fretting corrosion.
Modular interfaces are a central design feature of many total joint replacements and are often threaded or tapered. Geometric tolerancing, surface finishes, locking torques and assembly forces have a significant impact on the performance of these connections. Additionally, many of these mating surfaces are dissimilar materials which can create adverse conditions for corrosion, wear, and debris generation which can migrate into surrounding tissues.
Product fatigue testing can evaluate the overall mechanical performance of an implant in a laboratory environment, but additional testing and analysis are usually required to evaluate the corrosion conditions which could lead to in-vivo fracture. Such supplemental evaluations are not limited to orthopedics; electrochemical corrosion testing is often performed on other devices such as stents in the endovascular industry. Corresponding FDA guidance documents outline requirements for testing when stents overlap, or when considerations for tortuosity need to be made. This article will focus on fretting corrosion analyses that are commonly performed on shoulder, knee, and hip arthroplasty devices.
What are signs of fretting corrosion?
- Deformation of surfaces – The appearance of flattened, mushroomed, or otherwise deformed machining marks. Under magnification, even smooth surfaces have peaks and valleys. Even though the nominal stress between two surfaces may be small, the high spots are subject to asperity contact which can temporarily generate high stresses leading to the deformation of the peaks.
- Material Transfer – The removal of material from one surface generates debris that adheres to another surface. It can be mechanical in nature and generated due to micromotion between two surfaces, by either material (if different), or it can occur due to chemical reduction and oxidation processes which corrosively transfer material from one surface to another. It can also be a combination of these processes.
- Discoloration – Changes in color of the surfaces can occur due to fretting corrosion. Corrosion product is usually either black or green. Color change is dependent on material and fluid environment.
- Debris generation – Changes in color of the fluid environment and visible debris presence may be observed depending on the size and quantity of the debris generated. Clear solutions may still contain significant amounts of released material in the form of metal ions, which are quantifiable by inductively coupled plasma mass spectrometry (ICP-MS) or other inspection methods.
Regulatory Considerations
When submitting test results to a regulatory body such as the FDA, it is often required that fretting corrosion analysis be performed to evaluate the potential for failures and ion generation. This article outlines the methods and analyses that can be performed to ensure a thorough investigative testing program that addresses fretting and corrosion concerns, as well as providing objective evidence to demonstrate safe performance.
There are several test standards that were created to help evaluate fretting corrosion and debris generation.
- ASTM F1814 - Standard Guide for Evaluating Modular Hip and Knee Joint Components
- ASTM F1875 – Standard Practice for Fretting Corrosion Testing of Modular Implant Interfaces: Hip Femoral Head-Bore and Cone Taper Interface
- ASTM F897 - Standard Test Method for Measuring Fretting Corrosion of Osteosynthesis Plates and Screws
FDA guidance documents also provide recommendations for testing the modular connection, fretting, and corrosion testing alongside of the fatigue properties of the device.
What exactly should you be looking for? There are different ways to evaluate the modular connections, some quantitative and some qualitative. What is critical is that regardless of the analysis, adequate photographic evidence is provided to support the observations (or lack thereof). Regulatory bodies and manufacturers use this evidence to evaluate whether the device has undergone sufficient testing, what the overall risk is, and how the device compares to already approved devices on the market.
Test Method
Test specimens are cleaned and assembled according to the manufacturer’s surgical technique. Mechanical fatigue testing is then performed in a saline solution or bovine calf serum solution to simulate an in-vitro condition. The solution is encapsulated in a polymeric sleeve around the joint of interest. This is preferred as it limits any contamination from external factors (fixtures, cement, etc.), but it may not be feasible in all configurations. The test assembly can be fully exposed to the solution, but care should be taken to ensure that no external contaminants can enter the testing chamber, and fixturing should be designed to limit the number of materials that can interact with the test specimen. It is critical to tightly control the test parameters to eliminate any variables.
The 0.9% PBS saline solution is heated to 37ºC, and then mechanical fatigue loading is applied, usually in a cantilever bending condition. Once complete, the fluid is collected, the chamber is rinsed to ensure any particulate is captured, and the specimen is disassembled. Care should be taken to guarantee the surfaces are not compromised by tooling or post-test handling. Disassembly forces can be evaluated to help determine whether the applied torques or forces were adequate as well as identify if there is a locking issue. For example, a threaded connection may be completely loose depending on the wear and direction of loading.
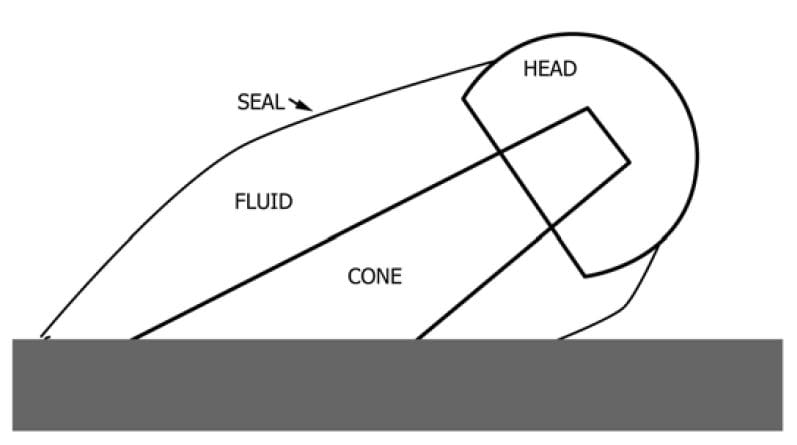
Types of Analysis:
Visual and Optical Inspection
Visual or optical inspection is the most common method for evaluating the effect of fretting corrosion during testing. Implants are documented pre-test with photographs and general observations. Observing the initial condition of the thread or taper will allow for a complete analysis. Once the specimens are disassembled, both visual and optical inspections should be performed. There are several approaches to evaluating the results.
JR Goldberg, et al. developed a scaling method referred to in his paper “A Multicenter Retrieval Study of the taper Interfaces of Modular Hip Prostheses.” This method has been used as the basis of many evaluation methods since 2002. Another paper authored by HS Hothi, et al. titled “The Reliability of a Scoring System for Corrosion and Fretting, and Its Relationship to Material Loss of Tapered, Modular Junctions of Retrieved Hip Implants” also uses this scale. Element uses a scale developed by Kevin Fricka, et al. titled “Metal-on-Metal Local Tissue Reaction Is Associated With Corrosion of the Head Taper Junction,” to evaluate the level of fretting corrosion.
A qualitative assessment of the interfacial fretting corrosion is conducted for the modular connections. The fretting corrosion, material transfer, and discoloration are evaluated using a scale of 1 to 5.
- 1 - No visual corrosion is observed.
- 2 - Less than 30% of engaged taper surface is discolored or dull.
- 3 - Greater than 30% of engaged taper surface is discolored dull, or less than 10% of engaged taper surface has black or dull gray debris, pitting, or etch marks.
- 4 - Greater than 10% but less than 50% of the engaged taper surface has black or dull gray debris, pitting, or etch marks.
- 5 - Greater than 50% of the engaged taper surface has black or dull gray debris, pitting, or etch marks.
Evaluations are performed on each quadrant of the surface, both proximally and distally, and are individually scored. Each connection also receives a composite score to evaluate the implant further. Ideally no or minimal fretting corrosion is observed; however, it is common for scores to vary on the different quadrants as loading can differ. A sample matrix is provided below:
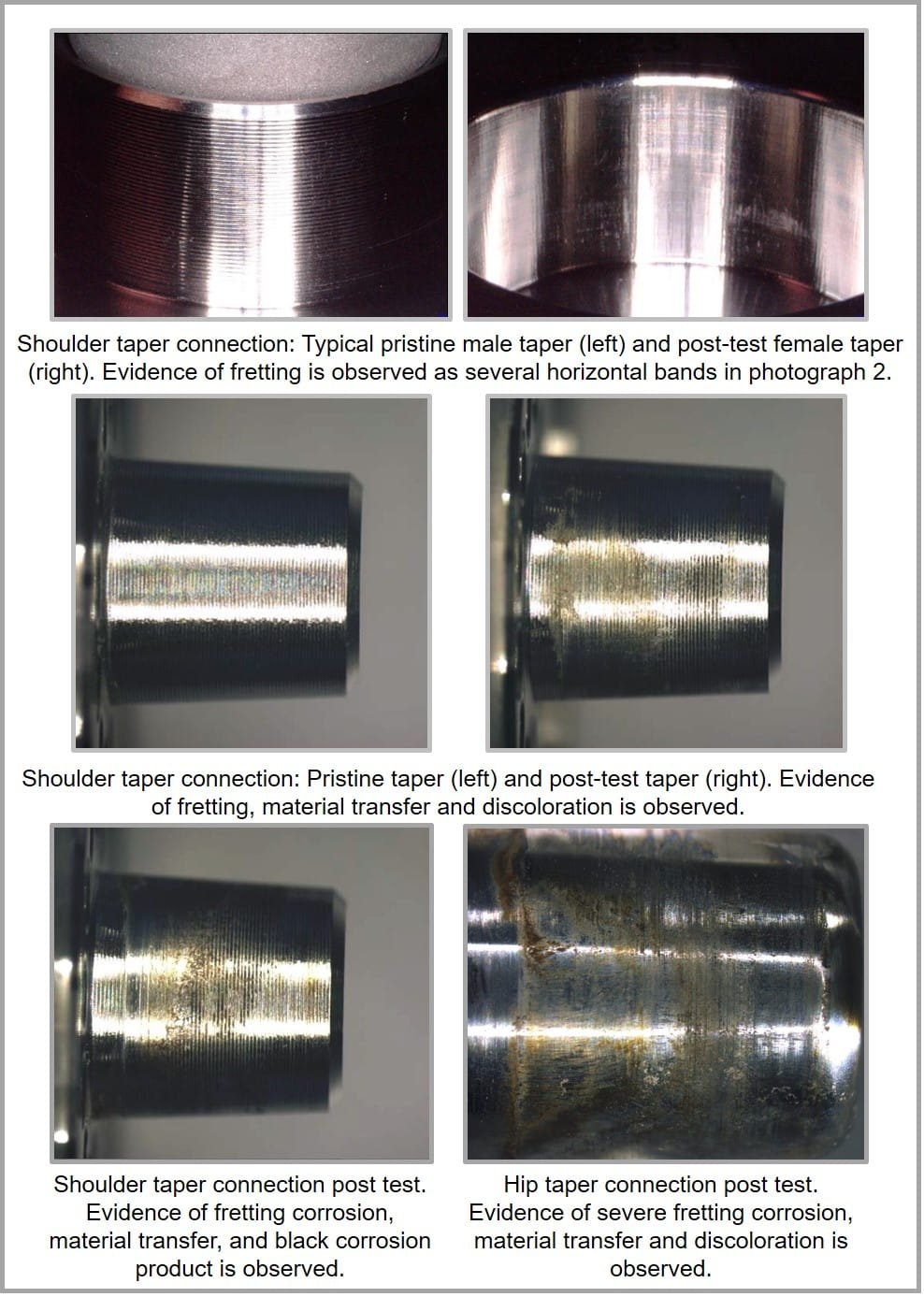
The amount of observed fretting corrosion, material transfer and discoloration is evaluated to provide as much evidence as possible. Several examples are listed below for reference on a shoulder and a hip implant. Advanced analysis can also be performed by using scanning electron microscopy (SEM), roundness measuring machines, or white light interferometry.
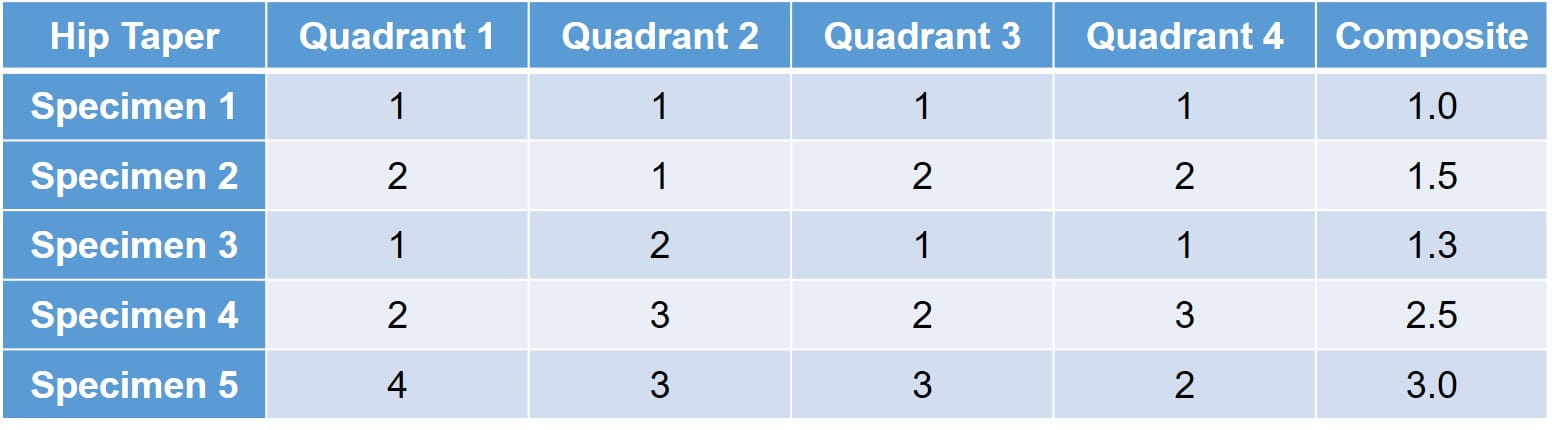
Mass Loss
Another test method for evaluating the amount of wear is to perform a mass loss analysis on each of the components. Element uses a scale that has 0.00001g resolution in order to evaluate whether sample mass has changed over the course of testing. The cleaning and drying process is critical to ensure an appropriate comparison, and a control specimen (not subject to mechanical fatigue) is sometimes used to mimic the environment. If the geometry is complex, salt precipitates as well as corrosive products can also form on the surfaces which need to be cleaned away for accurate measurement. Gloves should always be worn to prevent oils from your hands from contaminating the specimens. The mass loss analysis helps validate and quantify the visual findings. Ideally, no fretting corrosion will be optically observed and will be supported by mass loss analysis data.
Ion Analysis
The collected fluid can be analyzed for ions and wear debris using ICP-MS or other methods. Duplicates are often analyzed to observe the test repeatability.
Values below the detection limit are indicative of no or minimal ions supporting a positive test result and mitigating ion or free radical risks. The ions of interest need to be identified based on the material couple. Results can be compared to predicate data, previously-tested similar products, or to implant retrievals.
Conclusion
Corrosion is a significant concern for medical devices. The body is a corrosive environment and understanding both the impact the physiological environment has on the materials and the micro motion characteristics will help evaluate the safety of the implant. There are many types of electrochemical and corrosion interactions that are not covered in this article. However, the methods outlined aid in mitigating fretting corrosion risk in your device designs.
Adhering to these methods will help support a successful regulatory submission and thorough understanding of the fretting corrosion performance of your implant. In an ideal scenario, the data will show that there is no or minimal fretting corrosion, material transfer, or wear debris and be validated by the mass loss and ion analyses. In cases where adverse results are generated, comparison to literature, retrievals, or predicate side-by-side testing can be used to understand and predict overall implant performance.
The Element advantage
Bringing innovative medical device technology to the marketplace is a high‐risk venture. That’s why Element strives to provide absolute testing certainty by delivering accurate and reliable results for our clients’ medical device testing needs, from small, start-up ventures to large, established manufacturers. Our Engaged Experts are experienced in every stage of medical device testing, from test protocol development and prototype/feasibility trials to testing for 510(k), CE Marking, and other regulatory submissions.
As a comprehensive medical device testing partner, you’ll enjoy the benefit of a single-source supplier for all of your testing needs, from feasibility and R&D to product development and production quality control. We offer a full suite of medical device testing including:
- Mechanical testing
- Product qualification
- Material characterization
- Microbiological and chemical evaluation
- Packaging
- EMC/EMI testing
- Wireless Coexistence
- Leachables and Extractables
- Pharmaceutical
- Product electrical safety
At Element, our dedicated team of experts has many years of experience in a wide range of testing services for Class, I, II, and III medical devices to help you meet medical device regulations and ensure that every aspect of your medical device product is properly tested.
For more information about our fretting corrosion testing or other medical device services, contact us today.
Find related Resources